Flower Particles for Ultrasound-Guided Drug Delivery
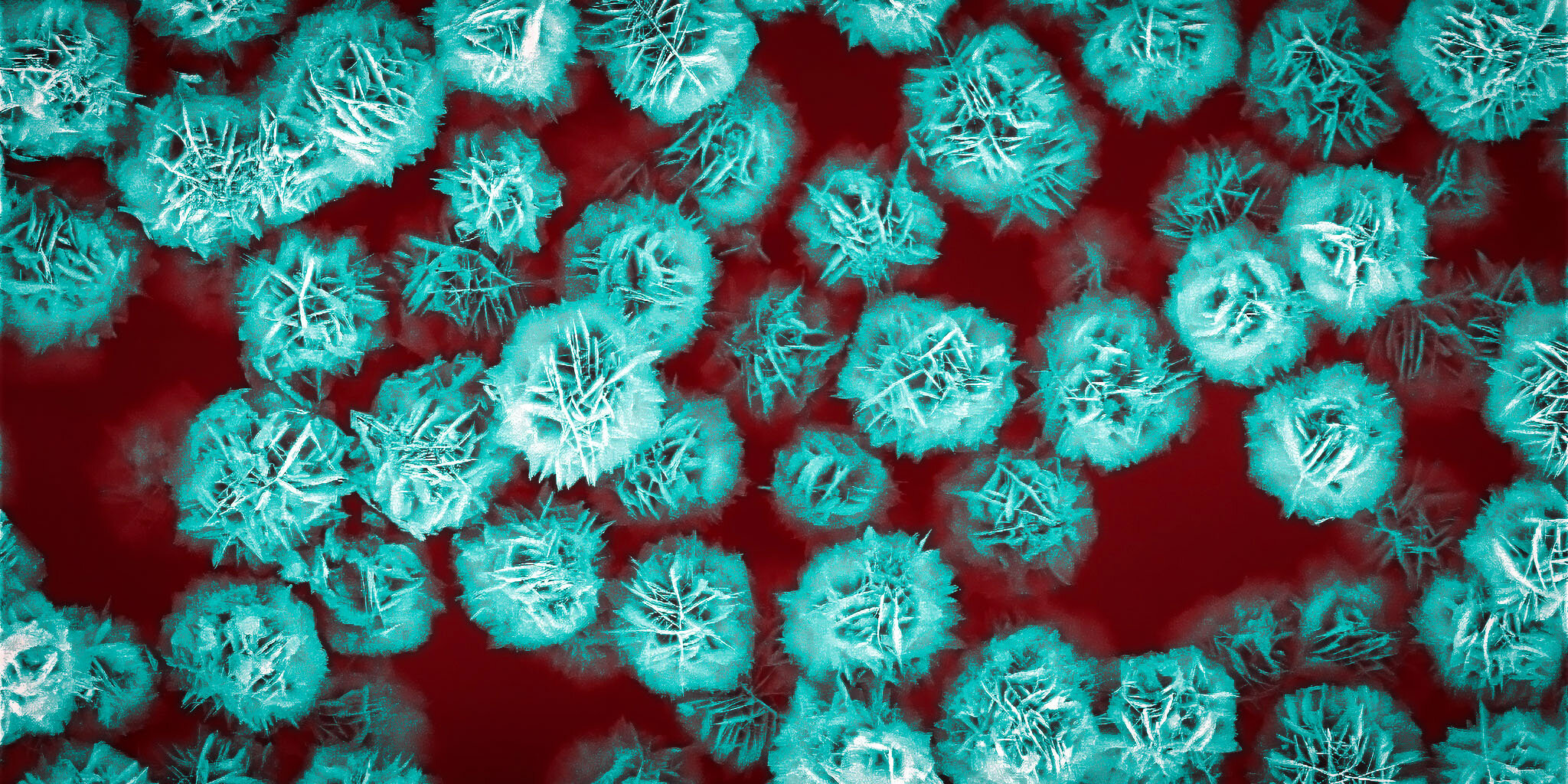
The Imperative of Precision: Revolutionizing Drug Delivery
The quest for targeted drug delivery represents a cornerstone of modern medical research, driven by the urgent need to enhance therapeutic efficacy while minimizing debilitating side effects. Imagine a world where potent medications could be directed with pinpoint accuracy to disease sites, leaving healthy tissues untouched. This ambition is particularly critical in the treatment of complex diseases like cancer, where conventional systemic therapies often inflict significant collateral damage on the patient's body. The ability to deliver therapeutic agents directly to a tumor, for instance, would not only improve treatment outcomes but also dramatically enhance the patient's quality of life. This fundamental challenge has spurred extensive investigation into innovative carrier mechanisms capable of navigating the intricate biological landscape of the human body.
Central to this endeavor is the identification and development of specialized carrier particles that can effectively bind and transport active pharmaceutical ingredients to their precise destinations. These particles must satisfy a demanding set of criteria to be clinically viable. Firstly, they must possess a high loading capacity, capable of carrying a substantial payload of the therapeutic substance to ensure effective treatment at the target site. Secondly, these carriers must be amenable to external guidance using non-invasive techniques, such as ultrasound, allowing clinicians to steer them through the complex network of blood vessels. Thirdly, and perhaps most crucially, their journey and ultimate delivery must be trackable in real-time using non-invasive imaging modalities. This verification is paramount to confirming successful drug deposition and ensuring therapeutic efficacy.
The convergence of these stringent requirements has historically presented a formidable challenge to researchers. Finding a single particulate system that seamlessly integrates high drug loading, efficient ultrasound guidance, and clear non-invasive tracking has proven elusive. However, recent groundbreaking research led by ETH Zurich has unveiled a novel and compelling class of particles that elegantly addresses all these critical criteria. These particles are not only functionally effective but also exhibit a visually striking morphology under microscopic examination, resembling delicate paper flowers or intricate desert roses. Their unique structure, composed of extremely thin petals that self-assemble into these floral configurations, offers a paradigm shift in the design of drug delivery vehicles.
The Bloom of Innovation: Introducing Flower-Like Microparticles
The innovative microparticles developed by the ETH Zurich team, ranging from 1 to 5 micrometers in diameter (slightly smaller than a red blood cell), represent a significant leap forward in the field of targeted drug delivery. Their distinctive flower-like shape is not merely aesthetically pleasing; it is the key to their exceptional functional properties. This carefully engineered morphology provides two primary and highly advantageous characteristics. Firstly, the intricate arrangement of the flower petals results in an extraordinarily large surface area relative to the particle's overall size. This maximized surface area is crucial for effectively binding and carrying a substantial quantity of therapeutic molecules, ensuring a potent and localized drug concentration at the target site.
Secondly, the unique architecture of the flower petals enables efficient interaction with external guidance and tracking mechanisms. The petals are designed to effectively scatter sound waves, making them readily amenable to manipulation and guidance through the bloodstream using focused ultrasound. Furthermore, these microparticles can be readily functionalized by coating them with specific molecules that absorb light, enabling their precise visualization and tracking within the body using advanced non-invasive imaging techniques such as optoacoustic imaging. This dual capability for both guidance and tracking provides clinicians with an unprecedented level of control and verification in the delivery of therapeutic agents, paving the way for more precise and effective treatments.
Functional Elegance: Unveiling the Advantages of Floral Design
The ingenious design of these flower-like microparticles confers significant functional advantages that surpass traditional approaches to drug delivery. The vast surface area created by the densely packed petals is a direct consequence of their intricate, three-dimensional arrangement. The nanoscale spaces, only a few nanometers wide, that exist between these petals effectively function as highly efficient pores. This unique porous structure allows the flower particles to absorb and retain exceptionally large quantities of therapeutically active substances, maximizing the drug payload that can be delivered to the targeted tissue or organ. This high loading capacity is critical for achieving therapeutic concentrations locally while minimizing the overall dosage required, thereby reducing the potential for systemic toxicity and unwanted side effects.
Beyond their impressive drug-loading capabilities, the flower particles exhibit remarkable responsiveness to external stimuli, enabling precise guidance and real-time tracking. The carefully engineered petals are designed to efficiently scatter sound waves, a property that is fundamental to their manipulation using focused ultrasound. This technique allows clinicians to non-invasively steer the particles through the bloodstream and concentrate them at a specific location within the body. Moreover, the option to coat the petals with light-absorbing molecules provides an alternative or complementary approach for visualization. Optoacoustic imaging, which detects the sound waves generated by these molecules when they absorb light, offers a highly sensitive and non-invasive method for tracking the particles' journey and confirming their successful arrival at the intended target site. This dual modality of guidance and tracking provides an unprecedented level of precision and control over the drug delivery process.
Superiority and Validation: Transcending Conventional Limitations
Previous research efforts in targeted drug delivery have extensively explored the use of tiny gas bubbles as transport vehicles that can be manipulated using ultrasound. While these gas bubbles have shown some promise, the flower-like microparticles developed by the ETH Zurich team offer a significant advantage: their ability to be loaded with substantially larger quantities of active pharmaceutical ingredients. This enhanced carrying capacity directly translates to a more potent therapeutic effect at the target site, potentially leading to improved treatment outcomes. The limitation of gas bubbles in terms of drug payload has long been a bottleneck in their clinical translation, a challenge that these novel solid microparticles effectively overcome.
The researchers rigorously validated the functionality of these flower particles through a series of compelling experiments. In controlled laboratory settings, they successfully demonstrated the ability to load the particles with a model cancer drug. Furthermore, to assess their in vivo behavior, the particles were carefully injected into the bloodstreams of living mice. Utilizing the precision of focused ultrasound technology, the researchers were able to actively guide and maintain the particles in a pre-determined position within the animals' circulatory systems. This remarkable feat was achieved despite the continuous and rapid flow of blood surrounding the particles, underscoring the effectiveness of the ultrasound guidance mechanism. This in vivo validation provides strong evidence for the potential of these microparticles to be effectively controlled and directed within a living organism.
Precision and Versatility: Tailoring the Technology for Impact
The ability to precisely control the movement and location of these flower particles using focused ultrasound represents a paradigm shift in drug delivery. This active control mechanism moves beyond the limitations of simply injecting drug-loaded particles and hoping they passively reach their intended target. Instead, clinicians can actively steer these microcarriers to specific areas of the body, such as tumors or blood clots that are obstructing vital vessels. This level of precision holds immense promise for revolutionizing the treatment of various diseases by delivering therapeutic agents directly to the pathological site, thereby maximizing efficacy and minimizing off-target effects. The researchers envision a future where this technology can be routinely used to deliver potent medications directly to cancerous growths, effectively destroying malignant cells while sparing surrounding healthy tissue. Similarly, they anticipate its application in dissolving dangerous blood clots that can lead to life-threatening conditions.
The versatility of this microparticle technology extends to the materials from which the flower-like structures can be fabricated. The researchers demonstrated that the underlying working principle is fundamentally based on the unique shape of the particles, rather than being solely dependent on a specific material composition. This opens up a wide range of possibilities for tailoring the particles to specific therapeutic applications and preferred imaging modalities. Depending on the intended use and the available imaging equipment, the particles can be made from a variety of biocompatible materials and can be further functionalized with different coatings. In their initial study, the researchers conducted detailed investigations using flower particles made of zinc oxide. They also successfully tested particles constructed from polyimide, a high-performance polymer, and even a composite material consisting of nickel and organic compounds. This material flexibility underscores the broad applicability and adaptability of this innovative drug delivery platform.
The Horizon of Hope: Towards Clinical Translation
The findings of this groundbreaking research pave the way for exciting future directions aimed at translating this innovative technology into tangible clinical benefits for patients. The immediate next step for the research team involves conducting more extensive animal studies to further refine the concept and optimize its performance in living organisms. These additional in vivo investigations will be crucial for gathering more comprehensive data on the safety, efficacy, and long-term behavior of the flower particles in a biological system. Following successful preclinical studies, the researchers are optimistic that this technology could eventually become a valuable tool for treating patients suffering from debilitating cardiovascular diseases, where targeted drug delivery to sites of vascular blockage could significantly improve outcomes and reduce the risk of serious complications.
Furthermore, the potential impact of these ultrasound-guided microscopic flower particles on cancer therapy is immense. The ability to deliver potent anti-cancer drugs directly to tumors, while sparing healthy tissues from the toxic effects of chemotherapy, could revolutionize cancer treatment paradigms. This targeted approach promises to not only improve patient survival rates but also significantly enhance their quality of life during and after treatment. By minimizing systemic exposure to cytotoxic drugs, this technology has the potential to reduce the debilitating side effects commonly associated with conventional chemotherapy, such as nausea, hair loss, and immunosuppression. The development of this technology represents a significant stride towards more personalized and effective cancer therapies, offering a beacon of hope for patients battling this complex disease. The promise of precisely delivering therapeutic agents to disease sites, guided by non-invasive ultrasound and tracked with advanced imaging, holds the key to a new era of medicine where treatments are more effective, less toxic, and ultimately, more beneficial for patients worldwide.